In Arizona and other western states, ranchers and land managers rely on thousands of miles of permanent wire fencing to manage livestock on extensive rangelands (Hayter, 1939). This type of fencing has led to improved rangeland conditions in many places by aiding in the application of grazing systems. However, wire fencing can fragment landscape connectivity, pose a risk to wildlife, is a major financial investment, and provides little to no flexibility to rapidly change pasture size, manipulate grazing distribution, or avoid areas of high use or sensitive habitat within a pasture (Holechek et al., 2011; Jakes et al., 2018). As a result, there are constraints on the use of permanent fences as a tool for managing riparian health, post-fire vegetation recovery, or improving livestock distribution. While electric fencing can be used to address some of these problems (Barnes and Howell, 2013), electric fencing can be hard to implement across large pastures and requires a significant time investment to setup and move.
Virtual fence (VF) technology is an emerging precision livestock management tool used to address these limitations and increase management flexibility and adaptive capacity to respond to changing environmental conditions as part of a larger grazing management system (di Virgilio et al., 2018; Lima et al., 2018; Trotter, 2010). As a management tool, VF uses invisible barriers, established by global positioning system (GPS) coordinates, that influence livestock movement with a combination of auditory and electrical cues (Antaya et al., 2024; Ehlert et al., 2024). Primary elements include: (1) a software interface to draw VF lines and the boundary zone on a digital map, which defines the grazing area and exclusion zone; (2) a GPS-enabled collar fitted around the circumference of an animal’s neck or other wearable device that contains technology to track livestock movement and deliver auditory and electrical cues to influence livestock distribution and (3) base stations and/or cellular towers to transmit and receive communications between the software and collars (Figure 1) (Antaya et al., 2024).
VF lines are created in VF software, which requires a digital map of an entire ranch or land management area. Ranchers and land managers may need to collect and verify Geographic Information Systems (GIS) data associated with their area to ensure accurate mapping. GIS data are the digital representation of physical features on Earth’s surface, such as roads, pasture or allotment boundaries, locations of waters, and landmarks. Accurate GIS data are highly beneficial for VF systems and may make it easier to apply precision livestock management across extensive rangelands.
This publication is part of a series on virtual fencing. Other titles in this series include:
- Basics of a Virtual Fencing System
- Training and Animal Welfare
- The Vital Role of High-Quality Data
- Exploring the Complexities and Challenges
- Strategies for Collar Management
- Collar Deployment Basics
- Economics of Virtual Fencing
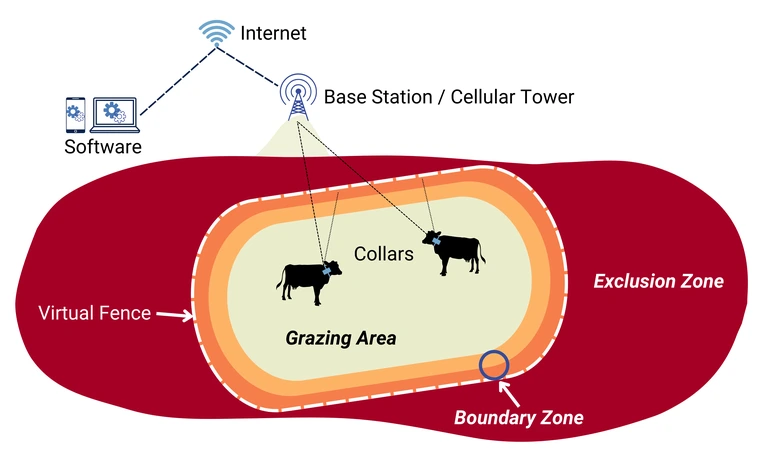
Figure 1: Conceptual model of hardware and software used to draw VF lines and define the grazing area, boundary zone, and exclusion zone
Quality GIS Data Reduces Risk
High-quality, accurate GIS data are highly beneficial for all pastures or grazing allotments where VF will be used, regardless of the acreage. Acceptable accuracy in GIS data is defined as data that are good enough to achieve your intended management outcome or reasonably collected based on your budget and available GPS device (e.g., smartphone, mapping-grade receiver, surveying-grade receiver) (Kennedy, 1996). GPS accuracy is often measured by horizontal position error, indicating how far (in feet or meters) the GPS point deviates from a precisely known location on the Earth. GIS data collected with a smartphone will have an average horizontal position error (i.e., accuracy) of approximately 33 ft (Merry and Bettinger, 2019). Generally, this should be an acceptable level of accuracy for most purposes related to VF. Physical infrastructure such as fence lines, gates, and seasonal and/ or permanent water features should be mapped within this acceptable level of accuracy. If the mapped infrastructure is not within this acceptable level of accuracy, the VF system may entrap an animal in an undesirable location, discourage an animal from moving through a grazing system, or unintentionally apply a VF that would prevent livestock from accessing essential resources such as water. This can result in an animal developing strategies that breakdown the VF system’s ability to successfully contain livestock within the grazing area (Mayer et al., In Press). To limit this potential risk, accurate GIS data are also recommended for other infrastructure and resources that may influence livestock movement such as: corrals, mineral and supplemental feeding stations, roads, grazing exclosures, cultural landmarks, and other landscape features. The seasonality of water features, such as dirt tanks, should be documented to ensure livestock have access to water when seasonal waters serve as the primary water source for livestock.
Finding and Collecting GIS Data
There are several methods to collect or acquire high-quality GIS data. On public lands, GIS data can be obtained from publicly available GIS data clearinghouses. The Arizona Geographic Information Council is Arizona’s primary source for geographic data and maintains GIS data resources for Arizona and other western states. The U.S. Forest Service (USFS) maintains an online collection of GIS data, including boundaries and ownership, natural resources, roads and trails, and other datasets. The Bureau of Land Management (BLM) also has an online collection of GIS data resources for BLM administered areas. (See Rangelands Gateway for links to GIS data resources: https://rangelandsgateway.org/virtual-fence). Selecting the appropriate GIS data may require technical skills to locate specific information within the geospatial data collections. In some cases, GIS file formats (e.g., files ending in .gpx, .shp, or .kml/.kmz) will need to be converted into a file format compatible with the VF software.
Data downloaded from GIS clearinghouses or obtained from agency sources should be verified to ensure accuracy. In some situations, satellite imagery (e.g., Google Earth) can be used to correct the GIS data that represent physical fence lines. However, some fence lines are not visible in satellite imagery or older imagery may not be up to date with current fence lines. It may be necessary to verify GIS data by ground truthing or collecting real-world GIS data on a smartphone or handheld GPS unit. Ground truthing confirms the data downloaded from third party sources are accurate. If inaccuracies or missing features are found, the GIS files will need to be edited. This may require GIS software skills on programs such as ESRI’s ArcGIS; Google Earth; or QGIS, a free and open-source GIS program. Some VF manufacturers may have additional resources to obtain GIS data for your area.
Private lands may lack geospatial resources on GIS clearinghouses and may require collection of GPS points to create the necessary GIS data. Cooperative Extension personnel may be able to assist with collecting GIS data on the ground, locating GIS data sources for your area, and digitizing paper maps into electronic files. Another option is to create your own GIS data with free-to-use GIS software (e.g., Google Earth, QGIS) which may provide satellite or aerial imagery with enough resolution to identify features (e.g., water tanks, gates, fence lines) within your grazing area. For example, you could identify water tanks from satellite imagery in Google Earth and mark the location of water tanks as points. Those points could be saved as a KML file and imported into the VF software, as most VF software allows you to import GIS data from common GIS data formats (e.g., KML). Additionally, data can be gathered in the field by collecting GPS points on a smartphone application (e.g., Gaia GPS) or handheld GPS unit (e.g., Garmin GPSMAP 67i). It may also be possible to create the GIS data in the VF software itself if the VF software has that option and provides satellite imagery with enough resolution for identifying features.
Addressing GPS Error
When implementing a VF system, consider GPS error. GPS works by satellites broadcasting radio signals from space, which are received by VF collars. Factors such as satellite geometry, signal blockage, atmospheric conditions, and receiver design affect the accuracy of the position determined by the GPS device (Thin et al., 2016). All GPS has some error associated with it, which can range from a few inches to thousands of feet depending on the device (Larsen et al., 1994; Villepique et al., 2008). A pilot study at the University of Arizona’s Santa Rita Experimental Range in southern Arizona used six Vence CattleRider™ ver. 2 rev. C collars (see Disclaimer) tied to posts approximately four feet off the ground for 30 days in basic tracking mode. In this mode, the VF collars are not near a VF and may have more GPS error than other operating modes. GPS points were collected every 30 minutes (Figure 2). The VF collars had an average horizontal position error (i.e., accuracy) of 37 ft, with a standard deviation of 157 ft (Figure 3) (Antaya et al., In Press). However, the five largest observations are not shown in Figure 3 and are very large: 409, 461, 4102, 8581, 9195 ft. These five largest observations represent 0.07% of instances but account for a large portion of the error, which would otherwise be smaller. GPS accuracy may improve over time. GPS accuracy will likely need to be periodically re-evaluated as new versions of VF collars are introduced, and new products enter the market.
In some situations, the GPS error alone may place an animal within a boundary zone, when the animal is, in reality, just outside the boundary zone. In this situation, the animal may unintentionally receive auditory and electrical cues. If this happens, livestock may be discouraged from moving through a grazing rotation or accessing water if the boundary zone is close to a gate or water. To limit unintended impacts of the GPS error, it is generally considered a best practice to avoid placing a VF within 100 ft of water, gates, and other essential infrastructure to give livestock ample space to safely access those areas. Additionally, avoid creating VFs with sharp corners (i.e., < 90 degrees) to allow the animals ample space to turn around before encountering a VF. Animals may get stuck in these sharp corners when surrounded by other animals, and GPS error may cause unintentional auditory and electrical cues (Mayer et al., In Press). High-quality, accurate GIS data combined with a buffer of at least 100 ft around essential infrastructure lessens the risk of animals unintentionally receiving cues. Unintentional cues can impact the system’s ability to effectively contain animals within the grazing area and may have consequences for animal welfare (Mayer et al., In Press).
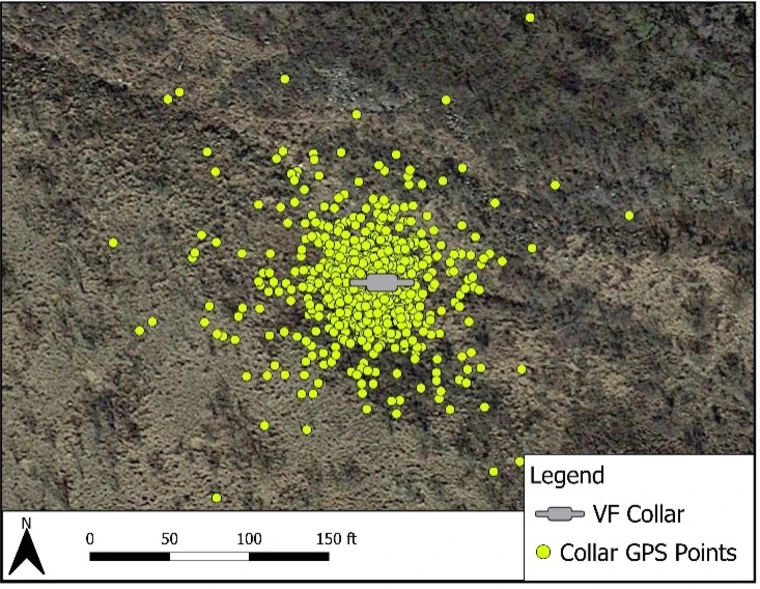
Figure 2: GPS points from a single stationary collar over a 30-day period.
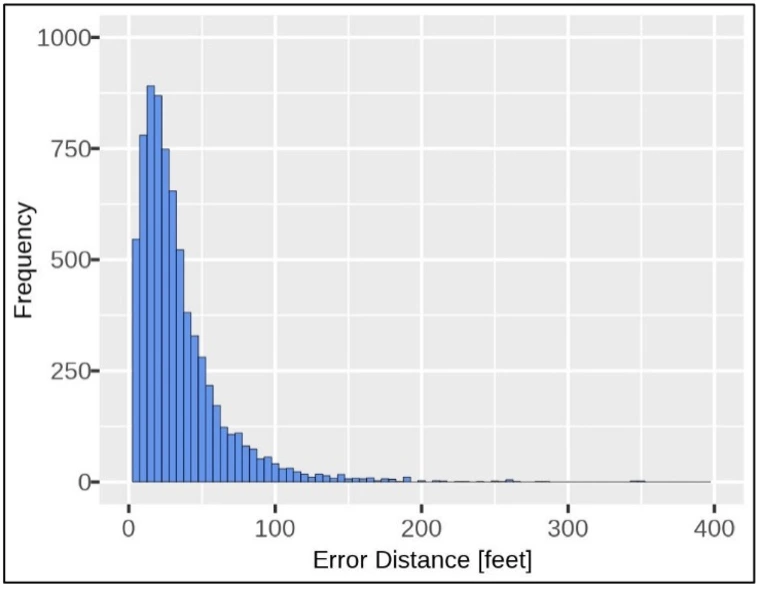
Figure 3: Distribution of GPS error from six stationary VF collars over 30 days. The
five largest observations (409, 461, 4102, 8581, 9195 ft) were removed to improve
readability.
Summary
A VF system uses invisible barriers to influence livestock movement with a combination of auditory and electrical cues. The success of a VF system hinges on the relationship between the physical landscape and a digital representation of that landscape to effectively manage livestock distribution. Translating on-the-ground knowledge to a digital representation in the VF software is an essential part to designing a VF. Important features such as waters, gates, and boundary fences should be accurately represented in the VF software to account for animals accessing essential resources. High-quality, accurate, and up-to-date GIS data are highly-beneficial for VF systems. Detailed GIS data, gathered personally or downloaded from GIS clearinghouses and verified for accuracy, ensures that VFs are properly positioned in the VF software relative to existing infrastructure. Local Cooperative Extension personnel may be able to assist with collecting GIS data or digitizing paper maps into electronic files. GIS data obtained from public sources are highly likely to have inaccuracies and missing features. When this happens the GIS files must be edited and errors corrected. Additionally, when using VF collars always consider GPS error. Avoid placing a VF within 100 ft of waters, gates, and other essential infrastructure, and avoid creating VF lines with sharp corners. This provides livestock ample space to safely access resources, while minimizing the risk of livestock unintentionally receiving auditory or electrical cues.
Additionally, there may be a minimum pasture size that is possible due to GPS error. A pasture that is smaller than the VF collar’s GPS accuracy is not recommended because an animal will be unable to avoid the boundary zone and will receive unintended cues. Follow the VF vendor’s guidelines on the minimum pasture size allowed. In some scenarios, such as in small pastures that are below the minimum pasture size requirement, VF may not be appropriate.
With high-quality GIS data, VFs can be accurately placed to protect sensitive habitat and ensure animal welfare. Further, accurate GIS data provides a more comprehensive understanding of the terrain and physical infrastructure across the landscape, which enables improved precision livestock management and real-time tracking of livestock. VFs can be easily adjusted to achieve management goals including encouraging livestock to move through a rotation, limiting access to ecologically sensitive areas, improving grazing distribution within a pasture, avoiding noxious species, and enabling targeted grazing strategies that reduce fuel loads or control invasive species (Figure 4). Ultimately, a foundation of high-quality, accurate GIS data are highly beneficial for implementing precision livestock management with VF systems.
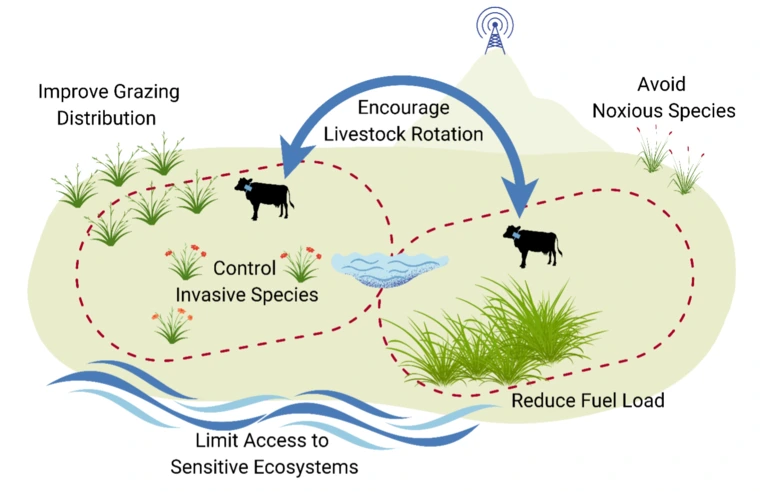
Figure 4: Conceptual model of the applications of VF.
Acknowledgements
This material is based upon work that is supported by the National Institute of Food and Agriculture, U.S. Department of Agriculture, under award number 2021- 38640-34695 through the Western Sustainable Agriculture Research and Education program under project number WPDP22-016. USDA is an equal opportunity employer and service provider. Any opinions, findings, conclusions, or recommendations expressed in this publication are those of the author(s) and do not necessarily reflect the view of the U.S. Department of Agriculture.
This work is supported by the AFRI Foundational and Applied Science Program: Inter- Disciplinary Engagement in Animal Systems (IDEAS) [award no. 2022-10726] from the USDA National Institute of Food and Agriculture.
Additional funding for the University of Arizona’s Virtual Fence program was provided by Arizona Cooperative Extension and the Marley Endowment for Sustainable Rangeland Stewardship.
For additional information about virtual fence technology, visit: https://rangelandsgateway.org/virtual-fence
References
Antaya, A., Dalke, A., Mayer, B., Blouin, C., Noelle, S., Blum, B., Beard, J.K., Ruyle, G., Lien, A., In Press. Challenges and Opportunities to Leverage Virtual Fence Data for Rangeland Management. Rangelands.
Antaya, A.M., Dalke, A., Mayer, B., Noelle, S., Beard, J., Blum, B., Ruyle, G., Lien, A., 2024. What is virtual fence? Basics of a virtual fencing system (No. az2079). University of Arizona Extension Publication az2079, Tucson, Arizona, USA.
Barnes, M., Howell, J., 2013. Multiple-Paddock Grazing Distributes Utilization Across Heterogeneous Mountain Landscapes. Rangelands 35, 52–61. https://doi.org/10.2111/RANGELANDS-D-13-00019.1
di Virgilio, A., Morales, J.M., Lambertucci, S.A., Shepard, E.L.C., Wilson, R.P., 2018. Multi-dimensional Precision Livestock Farming: a potential toolbox for sustainable rangeland management. PeerJ 6, e4867. https://doi.org/10.7717/peerj.4867
Ehlert, K.A., Brennan, J., Beard, J., Reuter, R., Menendez, H., Vandermark, L., Stephenson, M., Hoag, D., Meiman, P., O’Connor, R.C., Noelle, S., 2024. What’s in a name? Standardizing terminology for the enhancement of research, extension, and industry applications of virtual fence use on grazing livestock. Rangel. Ecol. Manag. 94, 199–206. https://doi.org/10.1016/j.rama.2024.03.004
Hayter, E.W., 1939. Barbed Wire Fencing: A Prairie Invention: Its Rise and Influence in the Western States. Agric. Hist. 13, 189–207.
Holechek, J.L., Pieper, R.D., Herbel, C.H., 2011. Range Management: Principles and Practices, 6th ed. Prentice Hall, Upper Saddle River, New Jersey.
Jakes, A.F., Jones, P.F., Paige, L.C., Seidler, R.G., Huijser, M.P., 2018. A fence runs through it: A call for greater attention to the influence of fences on wildlife and ecosystems. Biol. Conserv. 227, 310–318. https://doi.org/10.1016/j.biocon.2018.09.026
Kennedy, M., 1996. Global Positioning System and GIS, 1st ed. Ann Arbor Press, Inc., Chelsea, Michigan.
Larsen, W.E., Nielsen, G.A., Tyler, D.A., 1994. Precision navigation with GPS. Comput. Electron. Agric., Global Positioning Systems in Agriculture 11, 85–95. https://doi.org/10.1016/0168-1699(94)90054-X
Lima, E., Hopkins, T., Gurney, E., Shortall, O., Lovatt, F., Davies, P., Williamson, G., Kaler, J., 2018. Drivers for precision livestock technology adoption: A study of factors associated with adoption of electronic identification technology by commercial sheep farmers in England and Wales. PLOS ONE 13, e0190489. https://doi.org/10.1371/journal.pone.0190489
Mayer, B., Dalke, A., Antaya, A., Beard, J., Noelle, S., Lien, A., In Press. Foundations of Virtual Fencing: Training and Animal Welfare. University of Arizona Cooperative Extension.
Merry, K., Bettinger, P., 2019. Smartphone GPS accuracy study in an urban environment. PLOS ONE 14, e0219890. https://doi.org/10.1371/journal.pone.0219890
Thin, L.N., Ting, L.Y., Husna, N.A., Heikal Husin, M., 2016. GPS systems literature: inaccuracy factors and effective solutions. Int. J. Comput. Netw. Commun. 8, 123–131. https://doi.org/10.5121/ijcnc.2016.8211
Trotter, M., 2010. Precision agriculture for pasture, rangeland and livestock systems, in: Food Security from Sustainable Agriculture: Proceedings of the 15th Australian Agronomy Conference. Presented at the AAC 2010: 15th Australian Agronomy Conference: Food Security from Sustainable Agriculture, Australian Society of Agronomy Inc, Lincoln, New Zealand, pp. 1–6.
Villepique, J.T., Bleich, V.C., Pierce, B.M., Stephenson, T.R., Botta, R.A., Bowyer, R.T., 2008. Evaluating GPS collar error: a critical evaluation of Televilt POSREC-ScienceTM collars and a method for screening location data. Calif. Fish Game 94, 155–168.