Introduction
Due to its ubiquitous nature and prolific reproduction potential, powdery mildew (PM) is arguably the most economically important disease of wine and table grapes. The pathogen that is responsible for this disease, Erysyphe necator is a fungus, which if not held in check by chemical and/or cultural intervention, can compromise fruit quality and quantity in the vineyard. Although cultural practices such as shoot thinning and proper irrigation management are key to limiting infections, in most cases without the use of fungicides, PM would most likely be responsible for greater losses than any other single pathogen of grapes, worldwide. The disease was first described in North America (where it is believed to have evolved with North American Vitis species) in the early 19th century and was later observed in Europe, outside its native range (Gadoury et al., 2011). The introduction of PM caused severe crop losses in European wine grapes (Vitis vinifera L.), which were highly susceptible to the disease, and it was not long before the vast majority of grape growers in France were applying regular anti-microbial sulfur or copper treatments in order to keep the disease at bay. While the advancements in agricultural chemistry have led to greater consistency with regard to yields and quality, there are risks associated with the use of fungicides to the vineyard workforce, beneficial organisms of the vineyard, and the chemical’s own long-term efficacy due to the development of resistant strains of the fungus. These factors bring to the forefront the need to thoroughly understand the biology and disease ecology of E. necator, so that growers can develop an integrated pest management program that is not overly reliant on pesticides alone. In 2022, the University of Arizona conducted a Statewide Commercial Viticulture Needs Assessment to better understand the priorities of grape growers. The results stated that “Disease Management” was the third highest priority for growers with regard to Extension programming, and PM specifically was rated as the disease of greatest concern. While powdery mildew undoubtedly can be a problem in Arizona vineyards, the state’s dominant winter and summer bimodal precipitation pattern (Figure 1), coupled with high ambient temperatures and light intensity creates a unique management scenario.
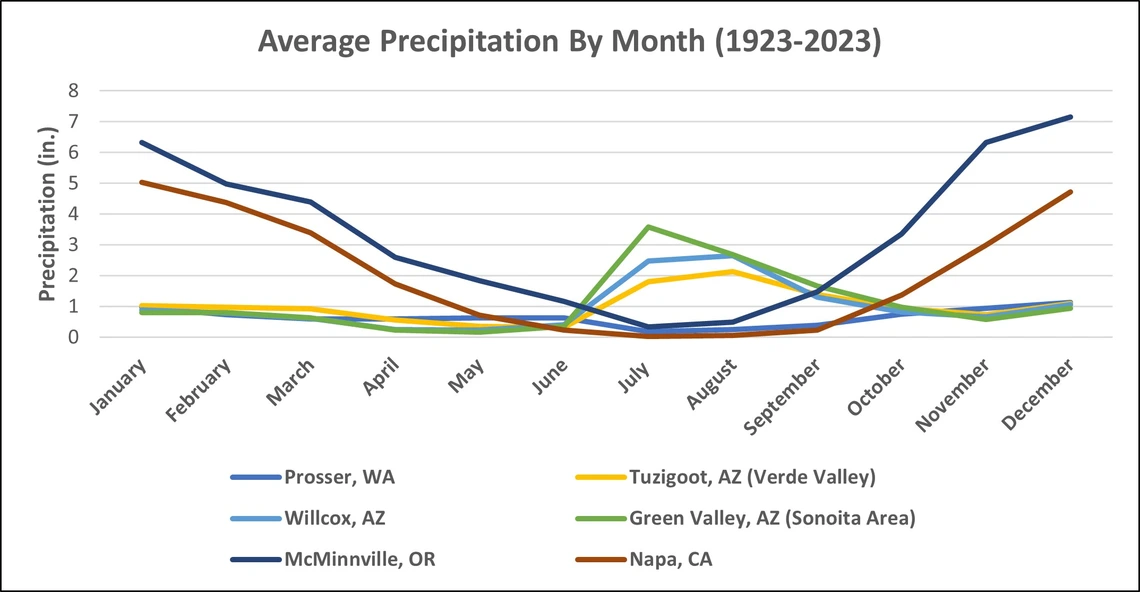
Figure 1. Average precipitation by site. Arizona precipitation patterns follow a bi-modal pattern, receiving the majority of its precipitation during the winter and summer months, and a relatively dry spring (National Weather Service Data).
Disease and Pathogen
Erysyphe necator is an obligate biotroph (it must obtain its nutrients from a living source) that reproduces in a polycyclic manner (Figure 2), which can lead to infections that are not detected until they have become a significant problem in the vineyard. Mature Chasmothecia (formerly called cleistothecia, Figure 3) are dispersed by winter rains from where they formed (on the green tissues of the vines) the previous year and are deposited in the vine’s bark or soil below (Gadoury and Pearson, 1988). In the following spring, when environmental conditions are favorable (average temperatures exceeding 50°F and a precipitation event, leaf-wetting irrigation, or sufficient dewpoint that deposits more than 0.1 inches of water), chasmothecia swell, break open and release ascospores, the sexual spores formed by genetic recombination (Moyer and O’Neal, 2013). This is referred to as a primary infection event. If an ascospore lands on a compatible substrate (green grape tissue), it will germinate (regardless of leaf wetness), attempt to attach itself, and penetrate the host’s cell wall (Gee et al., 2000, Micali et al., 2008). If attachment and penetration are successful, the fungus begins withdrawing nutrients and other resources from the grapevine without damaging the host’s cell membrane. This extraction is not limited to the plant cell in direct contact with the fungus, as nutrients throughout the host are mobilized to the site of attack. Upon a successful infection, the fungus will branch out on the plant’s surface in the form of hyphal colonies (the white/silver fuzz most often seen on leaves and fruit), using the vine’s resources to expand the hyphal network and initiate a secondary infection cycle referred to as “dispersal”.
Temperatures ranging between 50°F and 95°F are required for the proliferation of the secondary infection cycle, though E. Necator prefers temperatures that are regularly between 68°F and 85°F, relative humidity above 75% (free liquid water however, is not ideal) and low levels of direct solar radiation (Moyer and O’neal 2013). Dispersal involves the production of structures called conidiophores, which grow perpendicular to the powdery hyphal growth that covers the leaf surface and produce asexual spores called conidia, which are passively dispersed by mechanical pressure, wind, or convection currents. Upon landing on a suitable host, conidia germinate and begin the dispersal cycle once again (Glawe, 2008). It is also possible for E. necator to overwinter within dormant buds if those buds were infected while susceptible (4 to 6 weeks after bud break) in the prior year. It is unknown how often these “flag shoots” are observed in Arizona vineyards, so the importance of a robust scouting program cannot be over-emphasized.
The cycle begins again when chasmothecia form due to environmental conditions becoming unfavorable (low relative humidity, high levels of UV light, temperatures above 95°F, or plant tissue resistance) or at the point where an infection becomes severe enough that compatible mating types converge on the same tissue (Gadoury et al., 2011). Generally, chasmothecia are believed to then overwinter in the bark of the vine, though in warm areas where fall temperatures are mild such as southern Australia or the Mediterranean climates of Italy, it is possible for them to form by mid-summer, causing a second ascospore release and subsequent primary infection event within the same season (Gee, et al., 2000). It is unknown whether or not the vineyards of Arizona experience multiple generations of chasmothecia and ascospore release.
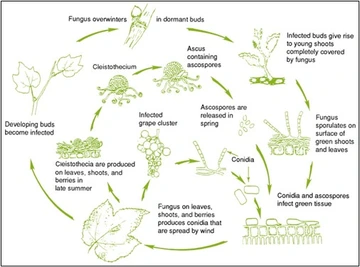
Figure 2. Drawing by R. Sticht (Kohlage) from Pearson and Goheen, 198
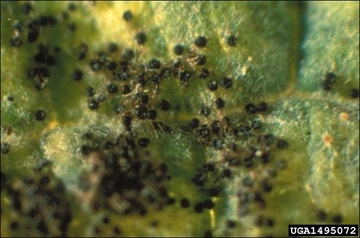
Figure 3. Mature Chasmothecia (formerly cleistothecia), the small, dark sexually reproductive survival structure of E. necator, formed on a leaf, most likely prior to deposition due to precipitation (photo: University of Georgia Plant Pathology , University of Georgia, Bugwood.org).
Signs and Symptoms
Due to the microscopic nature of the spores, symptoms of PM usually go undetected until they become more advanced. It is at this time that hyphal spread (the “powder”) and the secondary disease cycle begins (Figures 4 and 5). This is characterized by a shiny white or silver sheen (hyphae and conidiophores), though initial symptoms may appear as chlorotic spots which are challenging to diagnose. If the disease is allowed to progress, severe infections left unchecked can lead to the destruction of tissues and compromised fruit quality. Further, an infection by E. necator can leave fruit highly susceptible to infection by weaker pathogens such as Botrytis cinerea or the sour rot disease complex (yeasts and acetic acid-producing bacteria). Old infections are marked by “scarring” of the tissue (especially canes), which may appear as a spider web-like pattern in minor infections or large splotchy patterns in more severe cases (Figure 6). Due to the pathogenic and destructive nature of E. necator, severe foliar infection may reduce the photosynthetic capacity of the plant and the ripening of fruit, while also compromising the winter hardiness of vines in applicable areas.
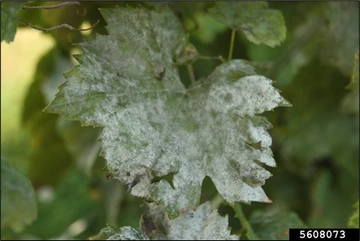
Figure 4. Grape leaf with hyphal infection by E. necator (photo: Gerald Holmes, Strawberry Center, Cal Poly San Luis Obispo, Bugwood.org).
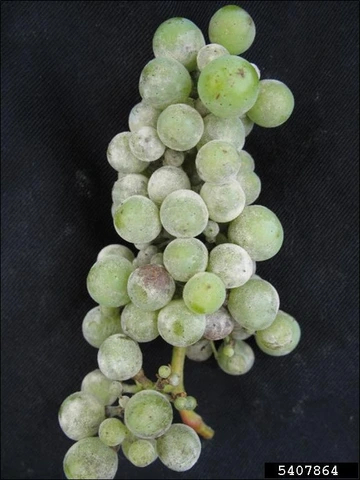
Figure 5. Powdery mildew infection on a grape cluster (photo: Julie Beale, University of Kentucky, Bugwood.org).
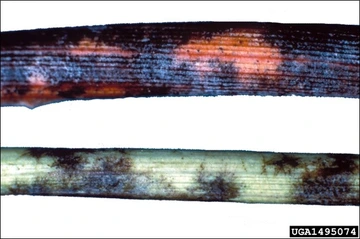
Figure 6. “Scaring” on cane and shoot tissue due to hyphal infection of E. necator (photo: University of Georgia Plant Pathology, University of Georgia, Bugwood.org).
Management in the Vineyard
Cultural Practices
Although some cultivars seem to be more susceptible to powdery mildew than others, complete resistance is uncommon amongst most cultivars of Vitis vinifera. The severity of a powdery mildew infection is highly dependent upon the environmental conditions, the inoculum level of the pathogen, and the phenological stage of the host. While the ambient macro and meso-climate conditions are generally those that are considered when reviewing the daily weather reports, the most important environmental conditions that influence disease development happen at the microclimate level. Within a vigorously growing grapevine canopy, temperatures are cooler and humidity levels higher than the area just outside of the canopy. This is due to plant transpiration (water evaporating from the leaf surface), the shaded nature of the canopy, and the vineyard’s physical ability to block the wind. Wine and table grape growers can manipulate canopy microclimate with cultural practices such as shoot-thinning and leaf removal, both of which open up canopies and expose their interiors to sunlight, heat, and ventilation (which lowers microclimate humidity). These practices may also facilitate effective spray coverage of applied fungicides. In areas where possible, irrigation management is also key to controlling canopy growth and transpiration rates. If an infection does prove successful, unfavorable environmental conditions will also drive the transition from dispersal (conidia spore release) to survival (chasmothecia initiation).
Fungicide Use
In some cases, cultural practices alone may be enough to deter grape PM epidemics, but due to the potential for crop loss, most growers worldwide rely on the use of chemical cover sprays in order to reduce infections. Historically, sulfur, copper, and oils have been used for both their prophylactic and eradicant qualities, but the 20th century introduced the broad use of synthetic fungicides, which are largely prophylactic and sometimes systemic (will move with the plant as it grows), allowing growers to stretch out spray application intervals. While sulfur is still used today (often in early season and organic production scenarios, today’s fungicide programs are dominated by the use of synthetic fungicides which interfere with the metabolism of the pathogen, often impacting its ability to germinate or proliferate hyphae. These synthetic fungicides belong to a number of different chemical classes, including benzimidazoles, the DMI and morpholine subgroups of the ergosterol biosynthesis inhibitors, strobilurin members of quinone-outside inhibitors (QoIs) and the succinate dehydration inhibitors (SDHIs). Quinoxyfen, proquinazid, metrafenone, and cyflufenamid are newer PM-specific fungicides that were recently introduced to the market.
A successful fungicide program begins before a primary infection event, relying on prevention and not eradication. After a primary ascospore-infection event (described above), it must be assumed that E. necator spores are present, and it is advised that growers use a registered fungicide at an interval that is appropriate for the level of pressure in the vineyard. Developing fruit clusters are most susceptible between approximately 3” of shoot growth (when inflorescences are initially exposed) and 3 to 4 weeks post fruit set (pea-sized berries) (Moyer and Grove, 2012, Ficke et al, 2002). This being said, berries in a single cluster develop asynchronously, and many growers will continue prophylactic applications until veraison, when the susceptibility threshold is more evident. After this period, there is no need to continue to treat the fruit, as it has acquired resistance to the pathogen (see ontogenic resistance below). Any new tissue, however (such as continued primary or lateral shoot growth), will continue to be susceptible to E. necator, so it is ideal to avoid an actively growing canopy after veraison.
Resistance Management
Due to high reproductive rates, its ability to infect under a wide range of climatic conditions, and its ready dispersal by air currents, E. necator has the propensity to develop resistance to many site-specific fungicides. Selection for resistant populations occurs when a site-specific product is:
- Used at below lethal application rates
- Used repeatedly in sequence
- Used numerous, non-sequential times in a season
There is probably already some resistance to a specific chemical mode of action among natural PM populations, and those individuals tend to survive after the application of that specific chemical. If a different chemistry is used at its maximum labeled rate during the next fungicide application, this small resistant sub-population is killed, and the resistance problem is most likely avoided. However, if the same chemistry is used repeatedly, the resistant sub-population is allowed to proliferate until the overall population is dominated by resistant individuals. This resistance effectively mutes the efficacy of the fungicide, giving growers one less tool to control disease. In order to combat this phenomenon, the Fungicide Resistance Action Committee (FRAC) has classified each product by its mode of action (Figure 7) and, more specifically, target site, which describes the biochemical process that is impacted by a product. These groups are represented by simple codes that separate chemical active ingredients based on mode of action. In order to limit the risks for pesticide resistance development, these groups should be rotated regularly throughout the season, while also avoiding overuse within a season, and/or low application rate treatments that some individuals may be able to survive. Furthermore, there is no known resistance to sulfur or oil products, though care must be taken not to apply at high temperatures.
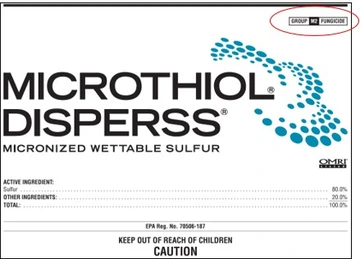
Figure 7. Fungicide label with FRAC group circled in red
Finally, in addition to canopy management and fungicide use, grapevine tissues eventually acquire resistance to E. necator. While it was previously believed that grape berries become resistant to PM at a specific brix level or at veraison, it is now widely accepted that host resistance is ontogenic, or “age-related”. Ontogenic resistance is the phenomenon by which plant tissues, as they mature, acquire resistance to infection by E. necator (Gadoury et al., 2011). In the case of berries, this resistance is believed to be acquired as early as 4 weeks past fruit set. However, rachis tissue does not readily acquire ontogenic resistance. Though the mechanisms are poorly understood, it is believed that plant resistance is acquired as tissues age, potentially through the development of preformed or physical biochemical barriers or the inducible synthesis of antifungal chemicals. Growers are advised to slow canopy growth after veraison, in order to halt the creation of new, susceptible tissue.
Due to the fact that Arizona has a unique climate in terms of precipitation patterns, heat accumulation and relative humidity, management of PM may be slightly different than other growing regions. Typically, Chasmothecia release ascospores in spring, when environmental conditions are favorable (as stated above), but Arizona is not generally characterized by wet spring events, and in some years, the region must wait until summer to experience significant precipitation, post-winter. Because of this, it may be possible for Arizona grape growers to hold-off on their initial fungicide applications, if they don’t experience the favorable environmental conditions necessary for a primary infection event. This being said, enough moisture from the deposition of dew can also trigger an infection event and growers must be confident that they can cover their vineyard with an approved fungicide before a spring rain event comes through. Temperatures above 95°F truncate infections and begin to kill the fungus, so if a vineyard has remained clean, once the summer heat has set in, it is unlikely that a secondary infection will proliferate. However, once the summer monsoon rains begin, cooling down temperatures and raising the relative humidity, there is potential for a latent secondary infection to begin on any actively growing tissues, or a primary infection event. By using their understanding of vineyard microclimate, proper fungicide management, and ontogenic resistance, growers can use cultural and chemical techniques more effectively in the ongoing battle against powdery mildew.
Cited Literature
Ficke, A., D.M. Gadoury, and R.C. Seem. 2002. Ontogenic resistance and plant disease management: A case study of grape powdery mildew. Phytopathology: New Frontiers in Plant Disease Losses and Disease Management Symposium. 92:6: 671-675
Gadoury, D.M. and R.C. Pearson. 1988. Initiation, development, dispersal, and survival of cleistothecia of Uncinula necator in New York vineyards. Ecology and Epidemiology. 78:11: 1413-1421.
Gadoury, D.M., L. Cadle-Davidson, W.F. Wilcox, I.B. Dry, R.C. Seem, and M.G. Milgroom. 2011. Grapevine powdery mildew (Erysiphe necator): a fascinating system for the study of the biology, ecology and epidemiology of an obligate biotroph. Molecular Plant Pathology. 13: 1:1-16
Glawe, D.A.. 2008. The powdery mildews: A review of the world’s most familiar (yet poorly known) plant pathogens. Annual Review of Plant Pathology. 46: 27-51
Gee, L.M., D.M. Strummer, D.M. Gadoury, L.T. Biggins, and E.S Scott. 2000. Maturation of cleistothecia of Uncinula necator (powdery mildew) and release of ascospores in southern Australia. Australian Journal of Grape and Wine Research. 6: 13-20.
Micali, C., Gollner, K., Humphry, M., Consonni, C., and Panstruga, R. 2008. The powdery mildew disease of Arabidopsis: A paradigm for the interaction between plants and biotrophic fungi. American Society of Plant Biologists: The Arabidopsis book: 1-19.
Moyer, M.M. and S.D. O’Neal (Editors). 2013. Field guide for integrated pest management in pacific northwest vineyards.
Moyer, M.M. and G. Grove. 2012. Powdery Mildew in Western Washington Commercial Grape Production: Biology and Disease Management. Washington State University Extension publication EM059E.