Monitoring soil moisture content is a critical aspect of effective irrigation scheduling. Maintaining optimal soil moisture levels is essential for plant growth and crop yield. Soil moisture monitoring can be accomplished through various methods, including the use of capacitance sensors that measure dielectric properties for volumetric water content assessments. Alternatively, traditional methods such as gravimetric sampling, primarily utilized in research, require weighing soil samples before and after drying to determine moisture content. These methods provide valuable insights for irrigation management, helping growers optimize water use and enhance crop productivity (Gu et al., 2020).
This publication reviews pertinent criteria for choosing a soil moisture sensor and appropriate technology that provides Long Range (LoRa) sensors or Long-Range Wide Area Network (LoRaWAN) equipment, Internet cloud, and related web application services for proper irrigation management. It compares soil moisture sensors and technology providers supplying equipment and software needed to manage irrigation through web-customized dashboard applications. At first, we examine the technical specifications outlined in datasheets to gain a thorough understanding of specific sensor technologies and their capabilities. Additionally, we provide extensive details regarding technical providers who offer support for managing sensor data, facilitating analysis, and presenting information through web applications. This valuable information enables growers to accurately determine optimal irrigation timings for their crops, with the possibility of automating the process. Irrigators typically receive notifications to manually execute irrigation tasks or through an automated system utilizing application programming interface (API) commands. Growers also have the option to create customized applications based on hypertext transfer protocol application programming interface (HTTP API) guidelines. Consequently, growers can efficiently oversee their irrigation management remotely via the Internet, utilizing either a computer or a mobile device.
Key insights from sensor datasheet
Analyzing a datasheet is crucial for individuals to make an informed choice when selecting a sensor. Datasheets are technical documents that describe the characteristics of a specific product. They are published by manufacturers and are usually found directly on the company’s website. Besides specific datasheets, the information might be available on the companies’ website, either through a labeled specifications tab or promotional brochures and videos. Table 1 shows eight important characteristics and the expected range of values, which can be used as a guide to determine what sensor capabilities are the most important to consider. Reviewing this information is well worth the effort and establishes the foundation towards purchasing the most suitable sensors.
Table 1. Main attributes of sensors included in datasheets.
Performance | Operation | Notes |
VMCa range: 0-100% | Temperature range: -20 to 50 °C | Measurement time: 10-100 msc |
Accuracy: 0.5-3% | VoIb 0.3-1.0 L | Sampling interval: 10-60 min |
Precision: 0.1-0.5% |
| Battery (sensor) life: 3-15 years |
a Volumetric Moisture Content, b Volume of Influence, and c Millisecond. |
Performance parameters
Prospective customers are primarily interested in the performance details of the soil moisture sensor when reviewing datasheets. Performance details include characteristics such as range, accuracy, and resolution. The common range of values for these performance parameters is shown in Table 1. All sensors that measure volumetric moisture or percentage should have a range from 0 to 100 % volumetric moisture content (VMC). There are other soil moisture sensors that operate on the principle of soil water tension (matric potential) such as the ones made by Sensoterra company. The accuracy and precision vary somewhat and are highly correlated to the type of sensor technology used for measurement. Table 2 shows a list of the common sensor technologies currently encountered in the market. This is described in more detail below.
Table 2. Example of sensors and adopted technologies.
Sensor | Web address | Technology | Depth Options |
HOBOnet Teros 12 | Capacitance | Single depth (4.5 in. length) | |
Decent Lab DL-SMTP-001 | https://www.decentlab.com/products/soil-moisture-and-temperature-profil… | Capacitance | multi-depth (24-in. depth) |
Dragino LSE01 | https://www.dragino.com/products/lora-lorawan-end-node/item/159-lse01.h… | Frequency Domain Reflectometry (FDR) | Single depth (7.7- in. depth) |
GroPoint 2625-ST | https://www.gropoint.com/products/soil-sensors/gropoint-profile | Time Domain Transmissivity (TDT) | multi-depth (up to 8 depths every 6 in.) |
Sensoterra single depth | Impedence (soil resistivity & capacitance) | Single depth (6-in, 12-in, 24-in, 36- in.) | |
AquaSpy® Legacy | Radio Frequency (RF) Permittivity | multi-depth (12 depths every 4 in.) | |
HOBOnet HS10 | Capacitance | Single depth (6.5- in.) | |
Pycno Terra Series | https://get.pycno.co/collections/pycno-core-all-in-one-soil-sensors/pro… | Capacitance | Multi-depth (3 depths up to 36 in. w/ 2 sensors each depth) |
Sensoterra multi depth | Impedence (soil resistivity & capacitance) | Multi-depth (6 depths up to 40 in.) |
Operation and lifetime
Soil moisture sensors should have a broad operating temperature range, allowing them to function effectively in various weather conditions. Therefore, it is important to observe these values to properly adapt the sensors according to the recommended ranges. Soil volume of influence (VoI), the extended range from the sensor where electromagnetic measurements are viable for evaluating the soil moisture, might be one of the most important operation parameters. The larger the volume, the better the sensor will perform. These volumes usually range from 0.026 – 0.26 gallons (0.1-1.0 liters). For example, the Meter T12 sensor has an unusually large volume of influence (0.26 gallons).
The lifetime of the battery is another important aspect to consider. Most sensors are equipped with batteries that usually have a lifetime ranging from 3 to 15 years, depending upon the battery type and the amount of power the sensor and long-range (LoRa) transmitter require. Thus, it is important to consider sensors with transmitter systems that use low power while they fulfill other required aspects. For instance, LoRa transmitters tend to use less power than other transmitter technologies. Some sensor systems such as AquaSpy Legacy, Pycno Terra, and Onset Meter sensors incorporate small solar panels in their systems (AquaSpy 2023, Pycno 2023, Onset 2023). The power supply options for the various sensors are outlined in Table 2. Since most of these sensor systems require low power, they contain one to two smaller batteries, such as C or D or lithium batteries. Another set of parameters that affect battery life are measurement time and sampling interval (ranges are shown in Table 1). Measurement times usually vary from 10-100 ms, and sampling intervals range from 10 minutes to 1.0 hr. They are factory-set but can be programmed by the operators themselves through the Internet of Things (IoT) server. The larger the sampling interval is, the longer the battery will last.
Other considerations
Growers must consider other decisive information when determining the suitable sensor for their needs. For instance, the communication range between the wireless LoRa transmitter and the base station or gateway is a significant factor to consider. Currently, the average distance is usually 1640 ft (0.5 km) from the sensor to a station, gateway, or repeater, depending on the above-ground installation height of LoRa transmitter. The stations or gateways usually broadcast up to 6.2 miles (10 km) or until it reaches the nearest cell tower. The cables attaching the RF transmitter to the sensor are usually 10-16 ft (3-5 m) long, which is normally documented in the datasheet. The warranty period for the sensor is also provided in the datasheet, which is usually one year.
When utilizing soil moisture sensors (capacitance- and resistance-based), soil texture and salinity levels should be considered. Capacitance-sensors, due to their sensitivity to soil dielectric properties, should be calibrated for specific soil types such as sandy, loamy, or clayey to optimize accuracy. Additionally, selecting capacitance-sensors designed for saline environments and regular calibration are crucial for mitigating the impact of soil salinity. Moreover, the efficacy of resistance-sensors may be influenced by soil texture and salinity. Hence, it is essential to consider soil texture in selecting and operating sensors, with regular calibration being a necessary step. For both types of sensors, it is advisable to emphasize regular maintenance, ensure proper installation depth, and follow the manufacturer’s guidelines for optimal accuracy. Also, calibration processes should be customized to the unique characteristics of the soil, ensuring precise and reliable soil moisture measurements for agricultural and environmental applications (Briciu-Burghina et al., 2022; Peddinti et al., 2020).
Companies usually provide the needed support for installing, troubleshooting, and maintaining the sensors and web software applications. This assistance can often be done remotely through a company’s sales or technical support agent at no charge. However, certain companies may impose service fees. Regarding calibration, most companies provide pre-calibrated sensors and suggest various calibration equations that could be applied to different soils.
Physical installation of sensors
In-situ soil moisture sensors can be installed at different depths within the root zone, horizontally or vertically, depending on their designs and measurement requirements (individual sensors or multi-depth probes). The most common range of installation depths varies from 6 inches (0.15 m) to 60 inches (1.5 m) to capture reliable soil moisture fluctuations. It is important to follow the installation steps of the sensors as recommended by manufacturers to achieve the most accurate results. For instance, when installing the sensors, minimum disturbance and removal of the soil should be considered and the condition of the soil near the sensor should be preserved to resemble the original soil. This is necessary to prevent faulty soil moisture or temperature measurements due to air gaps or the action of preferential flow that happens shortly after irrigation or rain events. In some cases, tools such as an auger are used to dig a hole where the sensors or probes should be inserted, which usually requires adding a soil slurry (self-mixing dirt and water) after installation to fill up the voids and prevent the air gaps.
Types of soil moisture sensors
Soil moisture sensors can be classified according to sensor technology, depth options and/or kinds of intended measurements (Table 2). Relevant information about most of the existing soil moisture sensors can be found online, thus it is recommended to visit the manufacturers’ web pages to do simple comparisons amongst all the sensors of interest. Capacitance, Time Domain Transmissivity (TDT) and Frequency Domain Reflectivity (FDR) are the primary technologies in the market. Other technologies such as radio frequency (RF) permittivity and resistivity-capacitance are available as well. Besides soil moisture, many sensors measure soil temperature as well as salinity, as electrical conductivity (EC). Also, soil sensors can cover single or multiple soil depths simultaneously. Table 3 provides an illustration of cost comparison between sensors, considering the packages they offer. However, for those prioritizing price, the preferred order of choice is outlined in Figure 1. Currently, single depth sensors usually range from $100 to $700 while the multi-depth ones are $600 to $1750. Although single depth sensors do not provide a substantial amount of information, they can still be sufficient for their intended purpose. For instance, soil moisture measurements within the effective root zone should provide adequate guidance for growers in planning the timing of their next irrigation.
There are many choices to consider when looking into purchasing multi-depth sensors. Detailed information of a larger soil profile helps with a better assessment of plant water-consumption needs and allows for accurate analysis and reliable irrigation management. Some companies offer the possibility to configure the number of measurement depths built into the sensor. For example, GroPoint and Pycno construct sensors where the customer can choose from one to several 6 – 12-inch segments, each containing soil moisture and temperature sensors (GroPoint 2023, Pycno 2023), whereas; other multi-depth probes are built with a fixed number of segments and sensors.
Table 3. Types of sensor measurements, connectivity, power supply and price.
Sensor | Measurement types | Connectivity | Power supply | Price |
HOBOnet Teros 12 | Soil moisture, soil temperature, and salinity | Chorded to data logger with wireless device (D) | Solar PV panel | $520 |
Decent Lab DL-SMTP-001 | Soil moisture and soil temperature | Chorded to LoRaWAN wireless device (A) | 2 alkaline C batteries (3 V total) | $1317 |
Dragino LSE01 | Soil moisture, soil temperature, and salinity | Chorded to LoRaWAN wireless device (A) | 4000 mAh Li-SOCl2 battery | $109 |
HOBOnet GroPoint multi-depth soil moisture sensor | Soil moisture and soil temperature | Chorded to data logger with wireless device (D) | Solar PV panel | $800 |
Sensoterra single depth | Soil moisture | Aboveground LoRaWAN wireless device (B) | Lithium battery - 3 V requirement | $252 - $294 |
AquaSpy® Legacy | Soil moisture, soil temperature, and salinity | Wireless connection (C) | Solar PV panel | $450 - $650 |
HOBOnet HS10 | Soil moisture | Chorded to data logger wireless device (D) | Solar PV panel | $320 |
Pycno Terra Series | Soil moisture and soil temperature (plug- ins for other sensors) | Wireless LoRa Peer to Peer connection (C) | Solar PV panel, 3500 mAh battery | $500 (10+ sensors) |
Sensoterra multi depth | Soil moisture and soil temperature | Aboveground LoRaWAN wireless (B) | Lithium battery - 3.6 V | $950 |
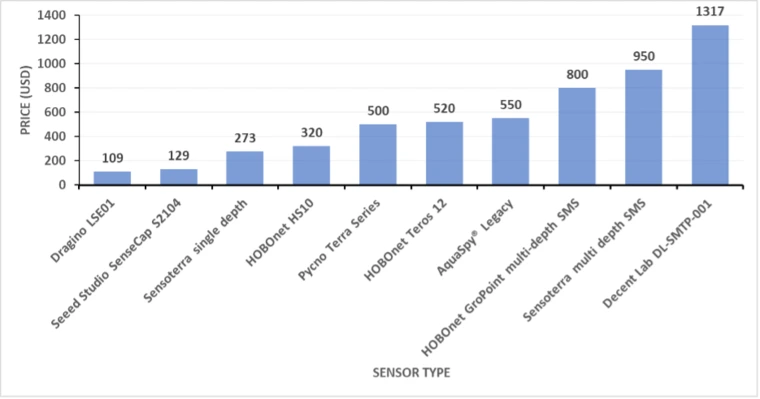
Figure 1: Average pricing (low to high) of soil moisture sensors as currently (Nov-2023) provided by different companies. Detailed packages are reported in Table 3.
Sensor connectivity designs and data transmission
Soil moisture sensors are built and configured in several designs. The diagram in Figure 2 illustrates some designs that are currently adopted. The most common sensor design is one that has a chorded connection between the sensor and LoRaWAN transmitter (A). There are also wireless configurations where the transmitter is connected within the sensor housing aboveground (B). Other systems contain the assembly that goes underground and then a solar power source and transmitter are above ground (C). Finally, some systems have underground sensors that are connected to dataloggers that also include transmitters (D).
Some soil moisture sensors have the capability to transmit data over extended distances via internet connectivity to cloud-based platforms. This is done through transmission and data communication technologies called LoRa and LoRaWAN. Long Range (LoRa) is a wireless modulation technique that can transmit data over longer distances compared to WiFi, cellular, and other technologies. Long Range (LoRa) devices are known to consume a low amount of power and that is why they are an attractive choice. Long Range Wide Area Network (LoRaWAN), a protocol built upon LoRa, is a software that determines how the sensors use the LoRa gateway to send and receive data. Practically, LoRaWAN is the wireless sensor technology utilized by soil moisture and other agricultural sensors for transmission and communication to the internet cloud using frequencies just below Gigahertz levels such as 915 MHz. Internet of Things (IoT) describes how individual electronic devices connect to each other and exchange data. This would include how the soil moisture sensors and gateways interact with one another. An IoT gateway is a network router that transfers data between the IoT sensors and the cloud. This system can transfer data that controls sensor actions such as sampling interval and measurement times.
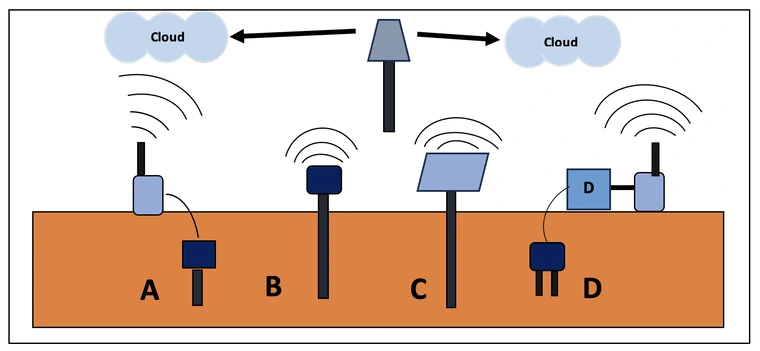
Figure 2. Types of connectivity for different soil moisture sensors and wireless LoRaWAN gateways and equipment.
Web application connectivity and functionality
Information collected from the sensors is transmitted through the IoT LoRaWAN gateway to the cloud that contains software applications to process the collected data. These applications are developed and owned by companies that supply the sensors, control the data, and provide related information to the customer. A succinct list of some technology providers that deliver such services is shown in Table 4, but more companies are available if a larger list is needed.
The list includes the cost of the wireless gateway equipment and data plans. The gateway equipment cost usually ranges between $399 - $2250. Repeaters (signal extenders) are often necessary as well and cost around $150 - $200. However, in many cases the cost is covered by the data plan, and sometimes the gateways are included with the sensors and so their cost is combined. Usually, the cost of data plan ranges from $150 - $350 per year or from $2 - $550 per sensor per year. The diagram in Figure 3 shows the type of software and services relevant to the sensors and their respective companies.
A wide variety of customized software services are available to help process, view data, and make decisions within a given threshold. For example, if the soil moisture levels drop below a management allowed depletion (MAD) level, a notification can be given, and an irrigation can be executed manually or automatically. Usually, a proprietary dashboard application is developed by each company to allow customers to view and download data or receive alerts. Moreover, the software can be viewed and utilized from android or iPhone systems. Certain providers have also created the option of controlling irrigation through API commands with options for the growers to control the sensor and irrigation management by using third party HTTP API applications. If the automatic irrigation scheduling is not needed.
Table 4. Example of Companies and sensors with their respective cost range for gateway equipment, data plans, and web application support.
Company and sensors | Wireless Connection Equipment | Data plans | Web application support |
Onset (HOBOnet sensors) (https://onsetcomp.com/) | $950 - $1250 Base stations (some have weather sensors), $150 repeaters | $150 - $350 per year for all sensors | Dashboard app to view data, give alerts, weather data, notifications, API for irrigation control |
Pycno (https://pycno.co) | $250 for IoT Gateway, $200 for weather station | $2 - $3 per sensor per year | Dashboard app to view data, give alerts weather data, notifications, API for irrigation control |
Decentlab (https://decentlab.com) | Have to buy gateway, repeaters, LoRaWAN provider | $294 per year for all sensors | Dashboard app to view data, give alerts, 3rd party HTTP API support |
AquaSpy (https://aquaspy.com) | Included with the sensors | Included with the sensors | Dashboard app to view data, give alerts, notifications, recommendations, HTTP API support |
Secure Infrastructure (https://infrastructure. securetool.company) | Sometimes wireless gateways are required, $299 weather station | $18 - $25 per year for each sensor | Dashboard app to view data, alerts, notifications, weather data, HTTP API support, full irrigation support |
Sensoterra (https://sensoterra.com) | Managed gateways $1250 - $2250 (ethernet or wireless) | Free first two years, $1.0 per sensor per year for all sensors | Dashboard app to view data, give alerts, notifications, HTTP API support, API for irrigation control |
Seeed Studio (https://seeedstudio.com) | Gateway $399, weather station $299 | Free first 180 days, pay as you go, recharge data plan ($0.99 1 month, $2.97 3 month, $11.88 12 month) | Dashboard app to view data, alerts, notifications, HTTP API support, API for irrigation control |
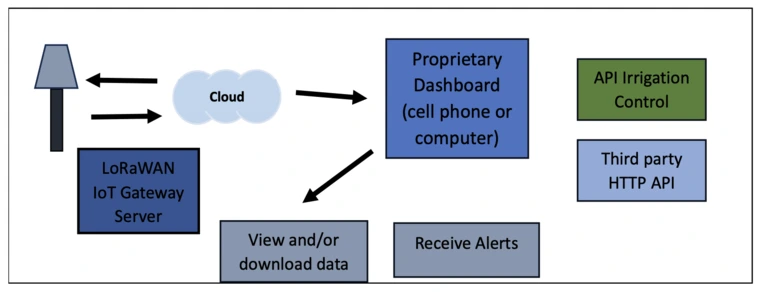
Figure 3. Diagram illustrating the functionality of the dashboard and other apps that are connected to the cloud from the IoT gateway server.
Conclusion
The set of criteria included in Table 4 should be considered by growers when determining the most suitable company to fulfil their requirements in terms of sensors and related equipment or services (IoT gateway, and weather information). Each of the aforementioned companies offer dashboard services, HTTP API, and irrigation support. It is important to highlight that the primary determining factor for many growers is the expense associated with soil moisture sensors, gateways, and data plans. Therefore, a pricing package which includes gateways cost with a free data plan, or a yearly cost might be more attractive than the packages offering separately priced equipment. However, sometimes connectivity (wired vs wireless), system designs (single vs multi-depth) and area size (small-scale vs large-scale) limit the choice, even when the price seems to be unreasonable. The diversity of equipment, data plans, and cost displayed in Table 4 offers a wide range of choice, but the right decision should not depend only on a single factor.
It is important to note that the sensors discussed in this paper serve as illustrative examples rather than a comprehensive representation of the market. Additionally, it is crucial to emphasize the necessity for future research to assess the accuracy, durability, and user-friendliness of various sensors, as these factors demand careful consideration. Furthermore, it is essential to clarify that the inclusion of specific names or trade mentions in the paper does not imply endorsement or support from the University of Arizona for the mentioned products.
References
AquaSpy, 2023a. Legacy soil moisture probe. https://aquaspy.com/legacy-soil-moisture-probe/
AquaSpy, 2023b. Personal communication with Steven Medieros
Briciu-Burghina, C., Zhou, J., Ali, M. I., & Regan, F. (2022). Demonstrating the Potential of a Low-Cost Soil Moisture Sensor Network. Sensors, 22(3). https://doi.org/10.3390/s22030987
GroPoint, 2023. GroPoint Profile. https://www.gropoint.com/products/soil-sensors/gropoint-profile
Gu, Z., Qi, Z., Burghate, R., Yuan, S., Jiao, X., & Xu, J. (2020). Irrigation Scheduling Approaches and Applications: A Review. Journal of Irrigation and Drainage Engineering, 146(6). https://doi.org/10.1061/(asce)ir.1943-4774.0001464
Meter group, 2023. Teros 12 Advanced Soil Moisture Sensors + Temperature and EC. https://www.metergroup.com/en/meter-environment/products/teros-12-soil-…
Onset, 2023a. HOBOnet T12 soil moisture/Temp/EC Sensor. https://www.onsetcomp.com/products/sensors/rxw-t12-xxx
Onset, 2023b. Personal communication with Eli Synder.
Onset, 2023c. HOBOnet multi-depth and single-depth sensors. https://www.onsetcomp.com/products/sensors/rxw-gpx-xxx
Peddinti, S. R., Hopmans, J. W., Najm, M. A., & Kisekka, I. (2020). Assessing effects of salinity on the performance of a low-cost wireless soil water sensor. Sensors (Switzerland), 20(24), 1–14. https://doi.org/10.3390/s20247041
Pycno, 2023. Terra all-in-one soil and ambient sensor. https://get.pycno.co/collections/pycno-core-all-in-one-soil-sensors/pro…
Seeed studios, 2023a. SenseCap outdoor gateway – LoRaWAN US915Hz. https://www.seeedstudio.com/LoRaWAN-Gateway-US915-p-4306.html
Seeed studios, 2023b. Personal communication with Lee
SensoTerra, 2023a. Single depth sensors – Multi-depth sensors. https://www.sensoterra.com/product.
SensoTerra, 2023b. Personal communication with Jessica Nuboer.